Concrete
Concrete is fireproof but not waterproof. It is heterogeneously composed mainly of coarse aggregate (stone), fine aggregate (sand) and hydrated cement as the binder. Strong in compressive but weak in tensile strength, reinforcing steel rods and/or prestressing tendons are included in the tensile zone; hence, the name “reinforced” and/or “prestressed” concrete.
Concrete may crack in the following ways:
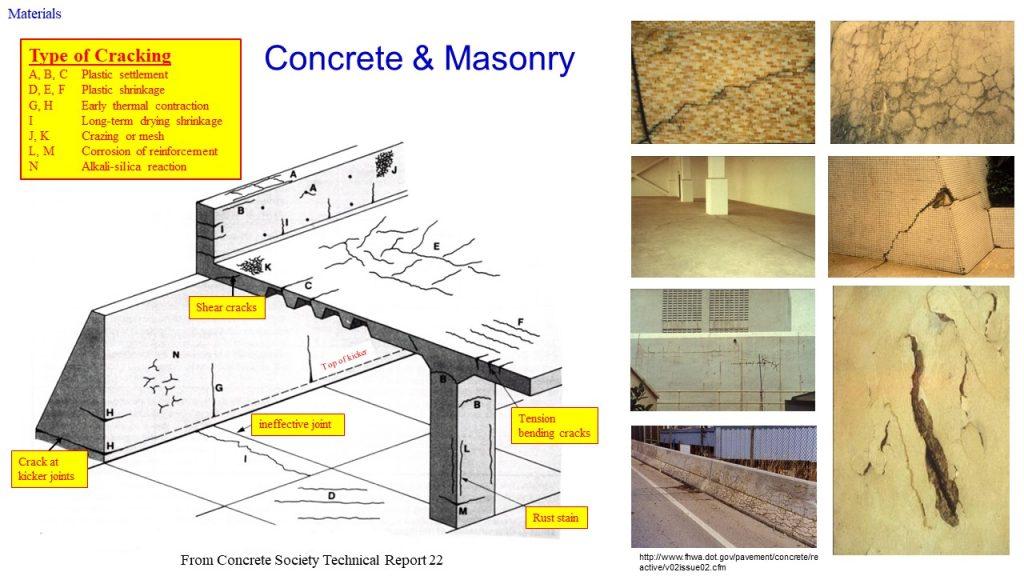
Structural Cracks
As concrete is weak in tensile/flexural strength, when a building is subjected to loads that exceed its designed capacity, due to e.g. an increase in live/dead loads, structural cracks may occur. With lack of provision of movement joints, cracks, in this case, may be resulted due to differential structural movement or differential settlement of foundation caused by lateral movement together. Uneven settlement of the soil and loading can result in differential settlement exceeding the tensile/flexural stress of the reinforced concrete causing the crack. Such differential settlement if continues, will result in the formation of a live crack and must be treated seriously. Such cracks appear more apparent on walls near the sinking foundation. The design and construction phases must pay special attention to strengthen the control of the uneven settlement of the foundation.
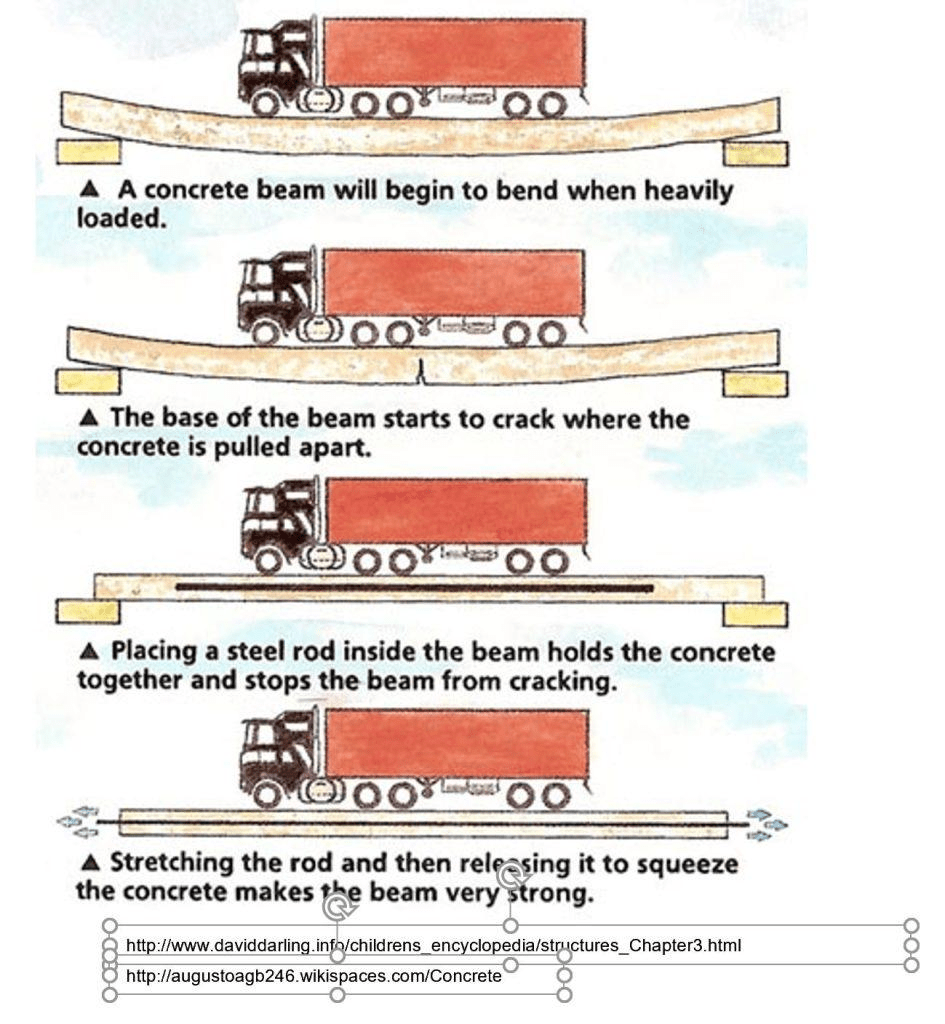
Non-Structural Cracks
Shrinkage Cracks
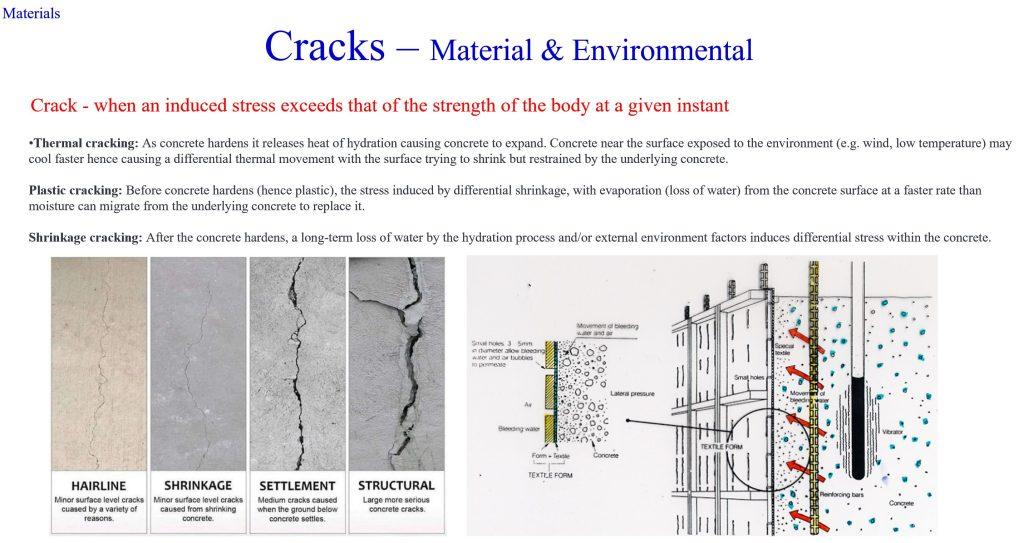

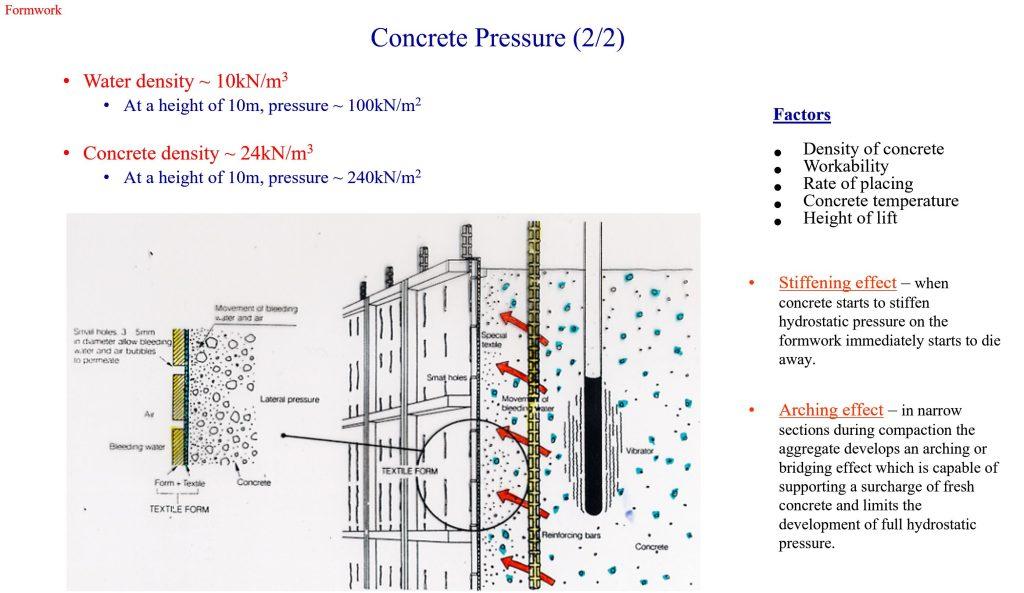
Concrete Spalling
Concrete contains micropores as a result of the migration of excess water during the hydration process. As the excess water dries off, they form channels or capillary pores. When subjected to water pressure, the pores allow the passage of water, resulting in leakage. When subjected to air pressure, the inner components, including the reinforcement, may be exposed to aggressive/corrosive agents, leading to cracks, delamination, spalling, water seepage, etc. Cement is made by roasting together crushed chalk (calcium carbonate) and clay slurry in giant steel kilns to a temperature of 1450°C. During this process, water and carbon dioxide escape, and the dry residue is crushed to form cement powder. This consists mainly of a mixture of calcium and aluminium silicates:
3CaCO3(s) + Al4Si4O10(OH)8(s) → Ca3SiO5(s) + Al4Si3O12 + 4H2O(g) + 3CO2(g)
During the roasting process, some calcium carbonate decomposes to calcium oxide but is not neutralised by the clay, so the cement, and the subsequent concrete, contains unreacted calcium oxide, a strong base:
CaCO3(s) → CaO(s) + CO2(g)
During hydration in the concreting process, or subsequent seepage of water, the calcium oxide dissolves in water to form calcium hydroxide solution (limewater):
CaO(s) + H2O(l) → Ca(OH)2(aq)
which is an alkaline with a pH as high as 13. This high alkalinity can be good, or bad:
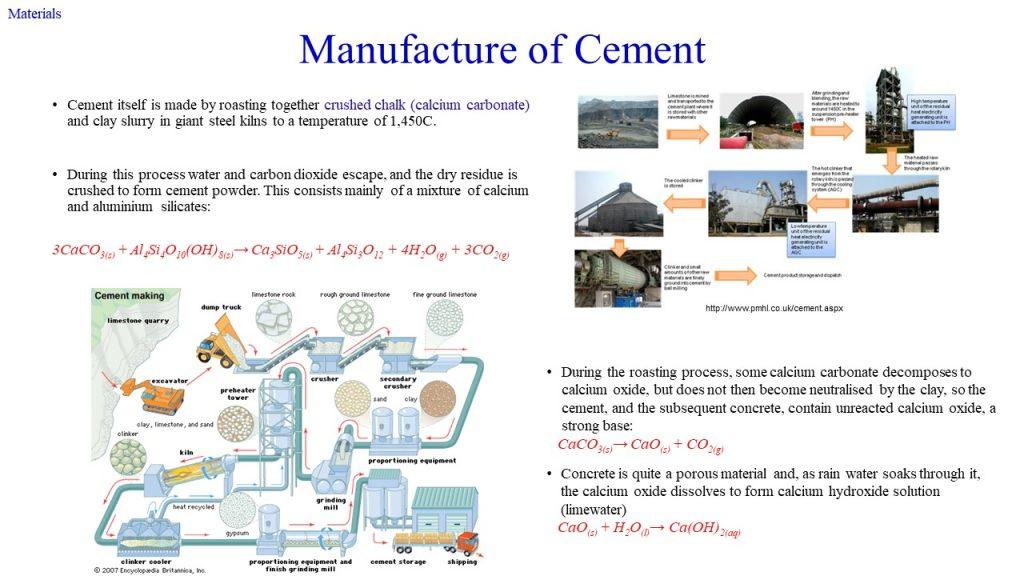
Alkali-silica reaction
The high alkalinity of Ca(OH)2 is bad in the case of an alkali–silica reaction (ASR) — a reaction between certain acidic aggregates with the alkali in the cement paste, resulting in an irreversible swell. During the ASR, a gel that swells as it draws water from the surrounding cement paste is formed. In absorbing water, these gels expand, thus inducing pressure and the subsequent cracking of the aggregate and surrounding paste.
The visual characteristics of an ASR include longitudinal cracks, map cracking, spalled surfaces, displacement of adjacent structural components, popouts, efflorescence, discolouration, and darkening. The usual technique for the identification of an ASR is (a) the use of a coloured dye field test as a preliminary test, followed by the examination of thin sections of concrete using petrographic microscopy, and (b) the use of a scanning electron microscopy (SEM), using a petrographic microscope, or a
scanning electron microscopy (SEM).
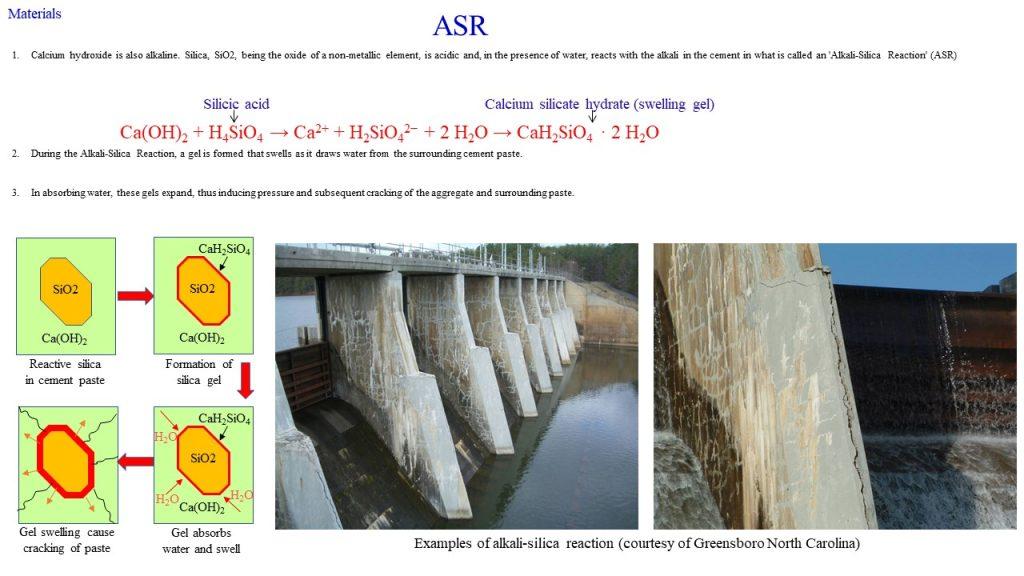
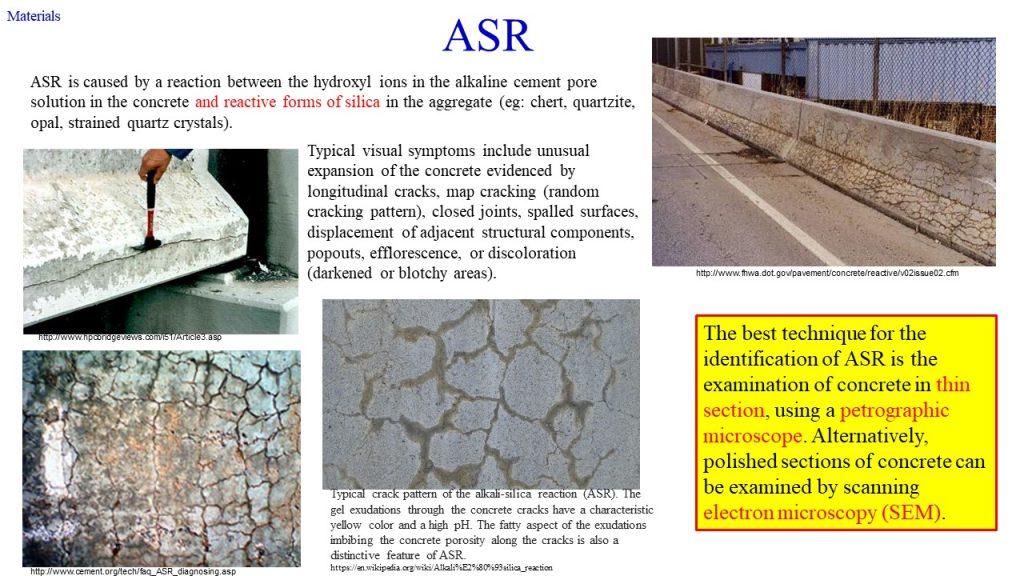
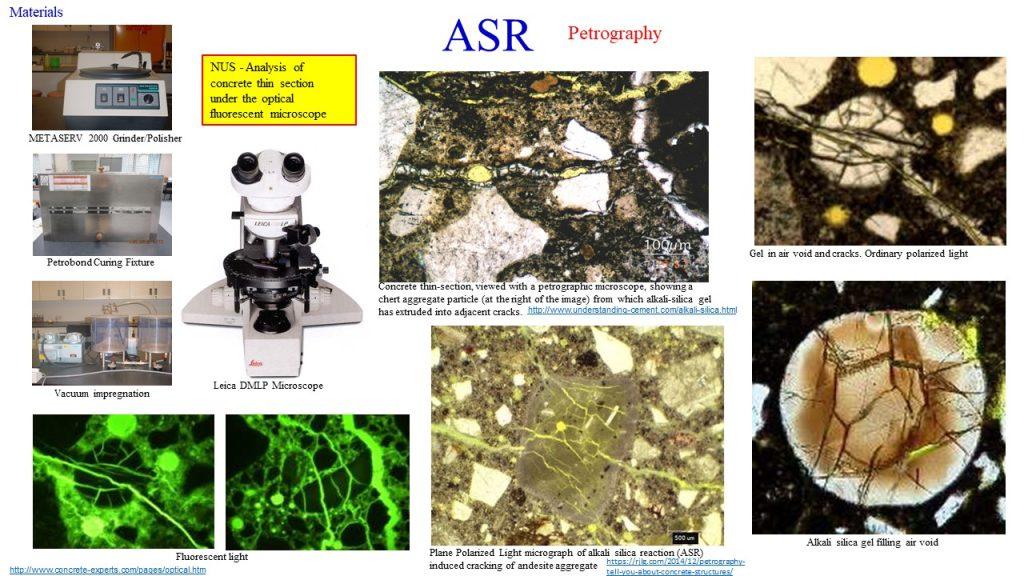
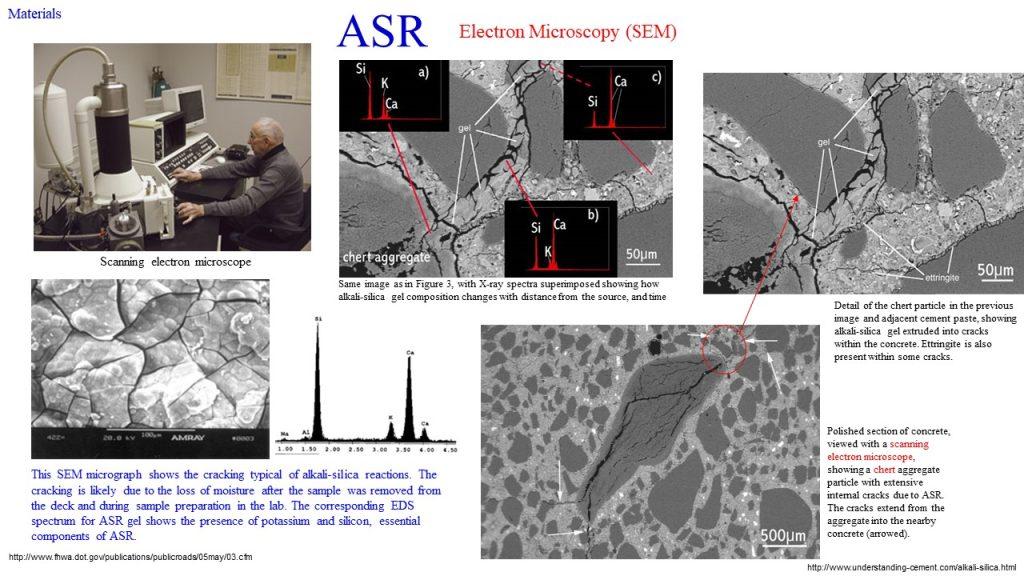
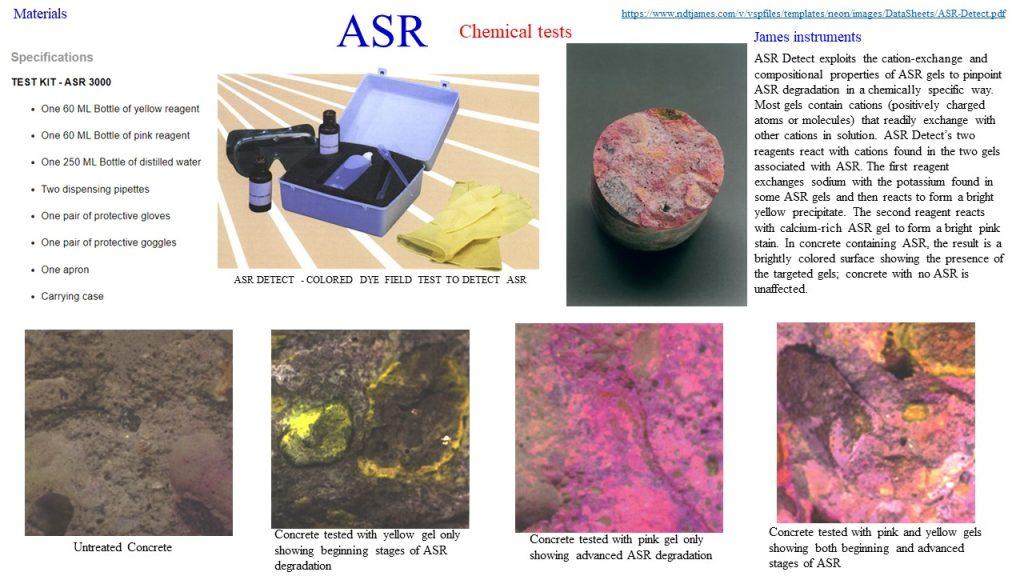
Reinforcement Corrosion
Corrosion is an electrochemical process, which can produce brownish colour deposits (rust) accompanied with increase in volume.
It represents a galvanic element – similar to a battery – producing an electric current, measurable as an electric field on the surface of the concrete. This potential field can be measured with an electrode known as half-cell. By making measurements over the whole surface, a distinction can be made between corroding and non-corroding locations. There are many scientific studies describing this method which has been known for more than 30 years.
As the rebar corrodes, rust forms and takes up more volume than the steel it replaces. (Figure 1) This exerts expansive forces on the concrete, which ultimately leads to cracking (Figure 2), spalling(Figure 3), and delamination of the concrete.
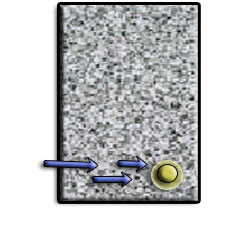
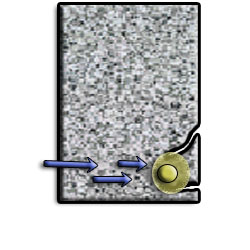
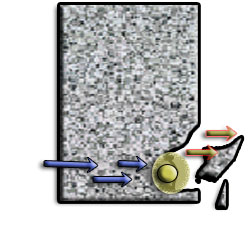
The 3 common reasons causing the reinforcement to corrode in RC are:
(1) Carbonation
(2) Cl– ion penetration
(2) Inadequate concrete cover (both quality and quantity)
The high alkalinity of Ca(OH)2 is good in the case of preventing reinforcement corrosion, as the calcium hydroxide solution forms a protective passivating layer around the reinforcement with an alkaline environment of pH up to 13. This alkalinity, however, can be destroyed by carbonation, where
Ca(OH)2 + CO2 → CaCO3 + H2O
or through the addition of chloride ions, which could enter concrete either (i) as an added component during mixing, deliberately included as an admixture, or (ii) as an external agent, e.g., seawater or breeze entering the already set concrete.
Chloride ion attack is another culprit responsible for pitting of reinforcement.
Efflorescence and calthemite formation have been discussed in various places. Other common forms of concrete durability issues include acid and sulphate attacks, freeze-thaw damage, shrinkage and creep, and cyclical thermal and moisture changes.
Corrosion – Concrete Reinforcement
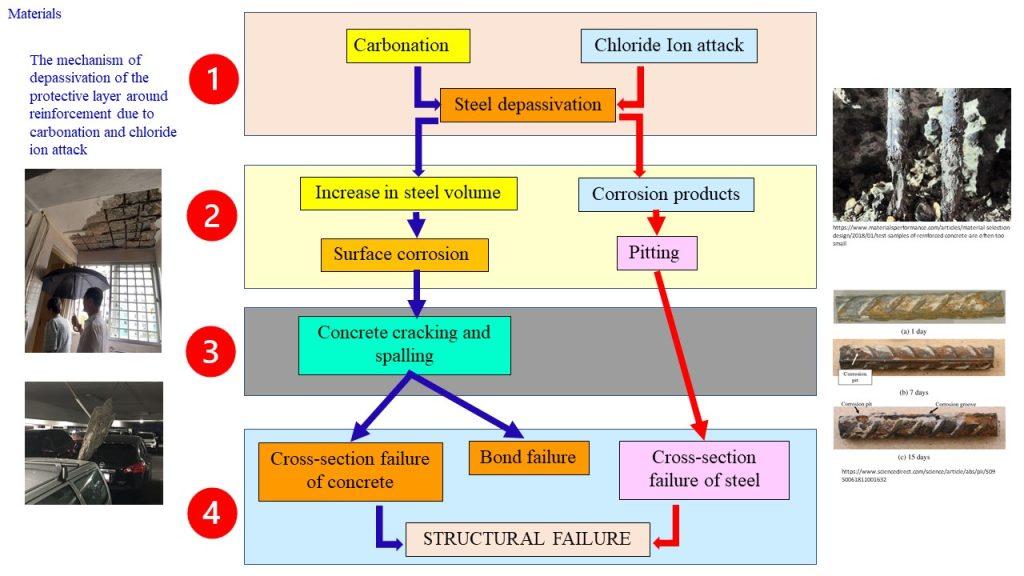
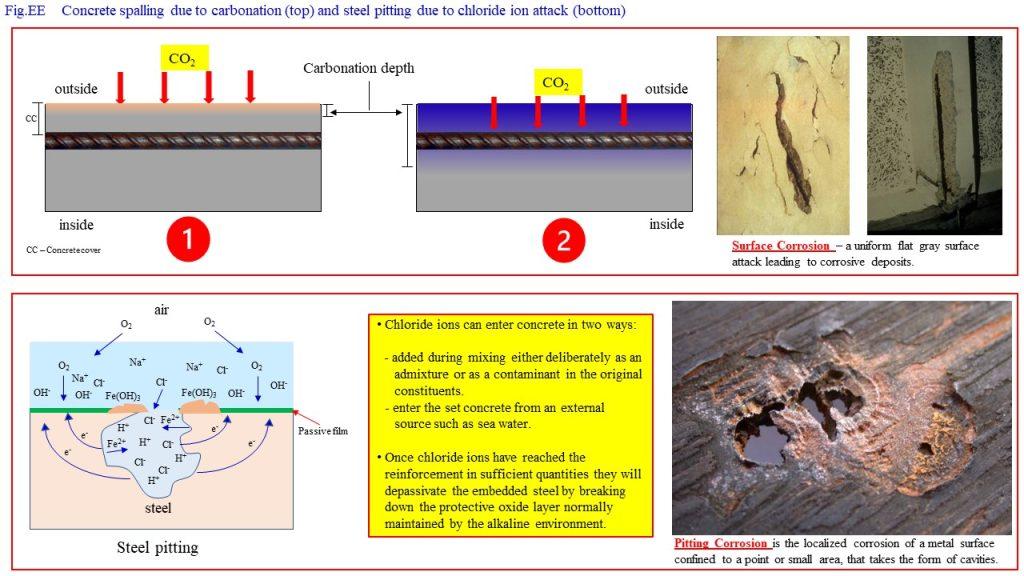
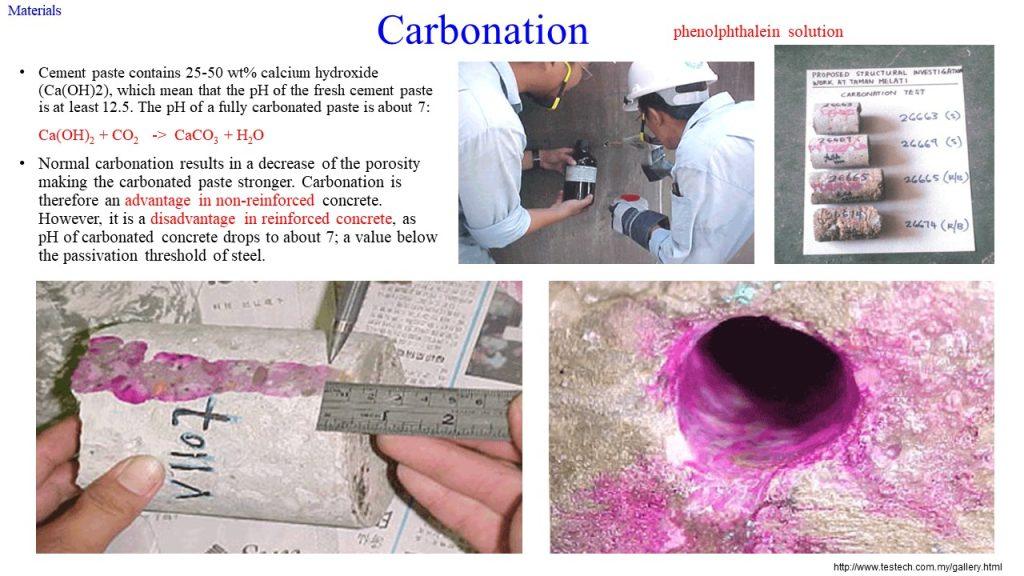
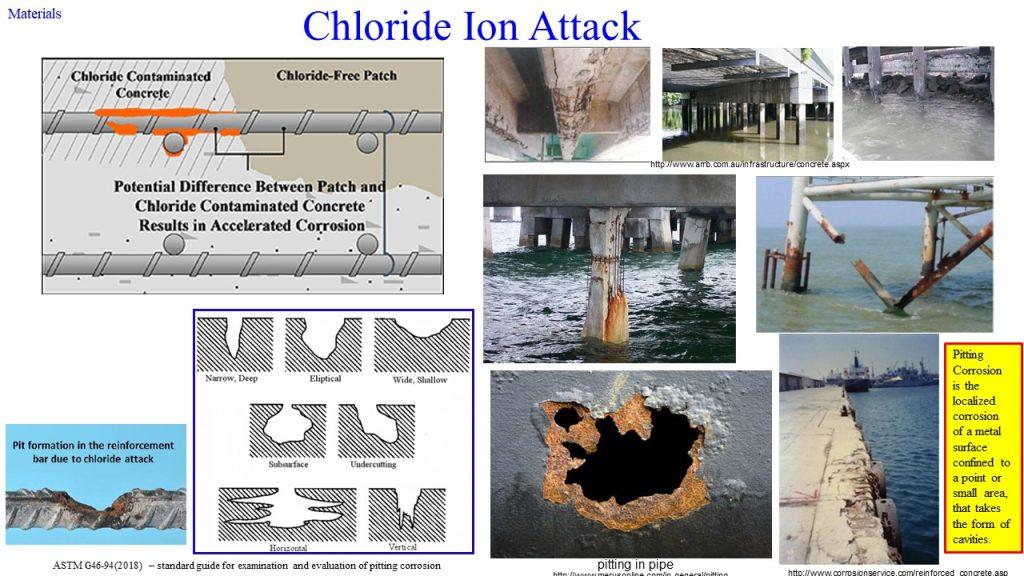
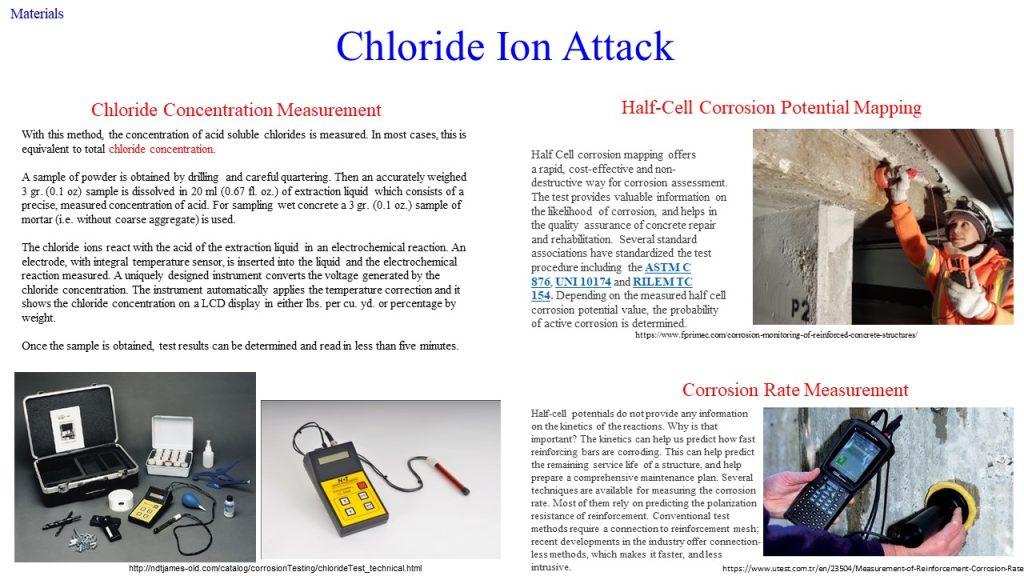
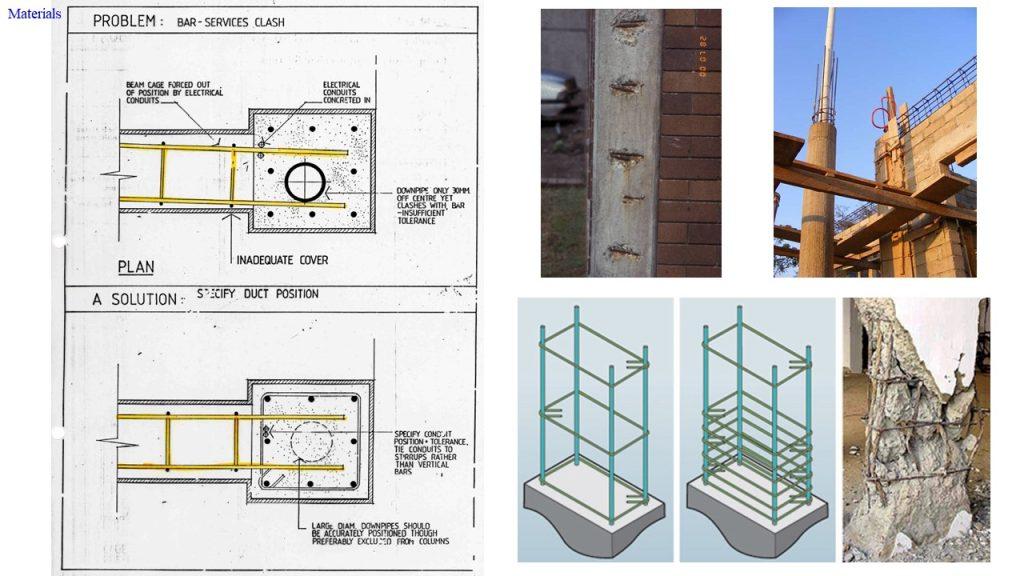
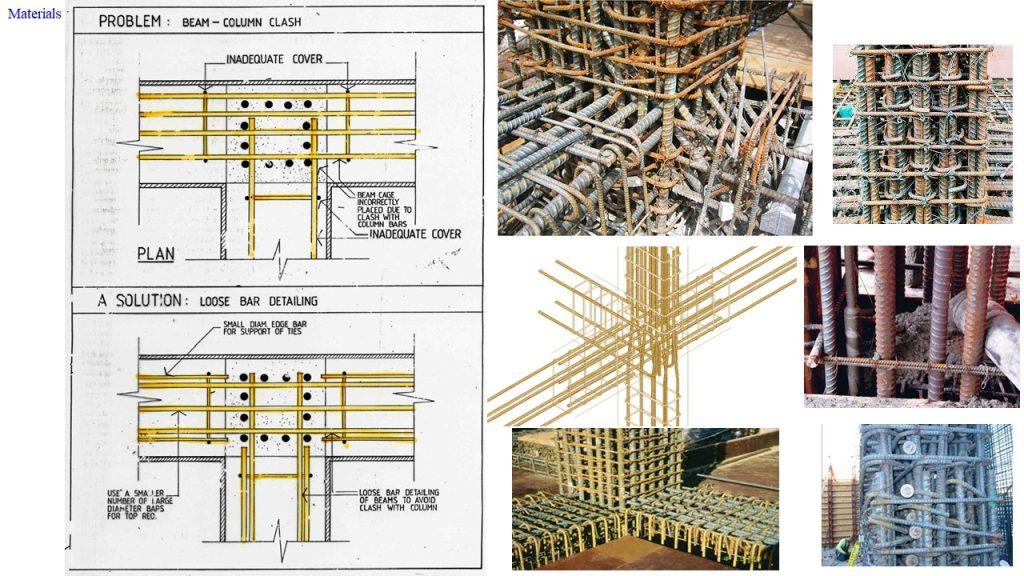
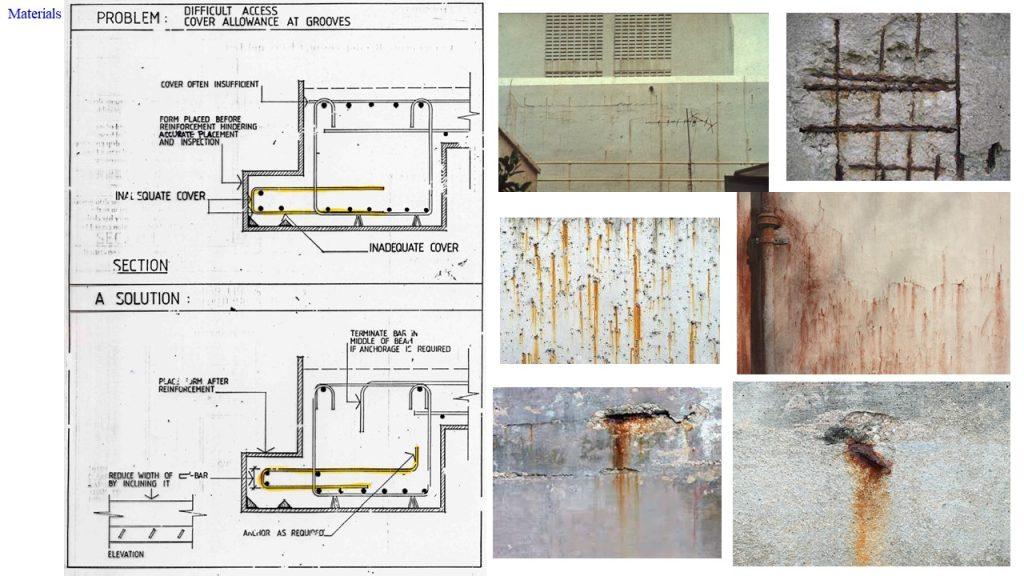